We report on proper motion measurements of the forward- and reverse shock regions of the supernova remnant Cassiopeia A (Cas A), including deceleration/acceleration measurements of the forward shock. The measurements combine 19 yr of observations with the Chandra X-ray Observatory, using the 4.2–6 keV continuum band, preferentially targeting X-ray synchrotron radiation. The average expansion rate is 0.218 ± 0.029% yr−1 for the forward shock, corresponding to a velocity of ≈5800 km s−1. The time derivative of the proper motions indicates deceleration in the east, and an acceleration up to 1.1 × 10−4 yr−2 in the western part. The reverse shock moves outward in the east, but in the west it moves toward the center with an expansion rate of −0.0225 ± 0.0007 % yr−1, corresponding to −1884 ± 17 km s−1. In the west, the reverse shock velocity in the ejecta frame is ≳3000 km s−1, peaking at ∼8000 km s−1, explaining the presence of X-ray synchrotron emitting filaments there. The backward motion of the reverse shock can be explained by either a scenario in which the forward shock encountered a partial, dense, wind shell, or one in which the shock transgressed initially through a lopsided cavity, created during a brief Wolf–Rayet star phase. Both scenarios are consistent with the local acceleration of the forward shock. Finally we report on the proper motion of the northeastern jet, using both the X-ray continuum band, and the Si xiii K-line emission band. We find expansion rates of, respectively, 0.21% and 0.24% yr−1, corresponding to velocities at the tip of the X-ray jet of 7830–9200 km s−1.
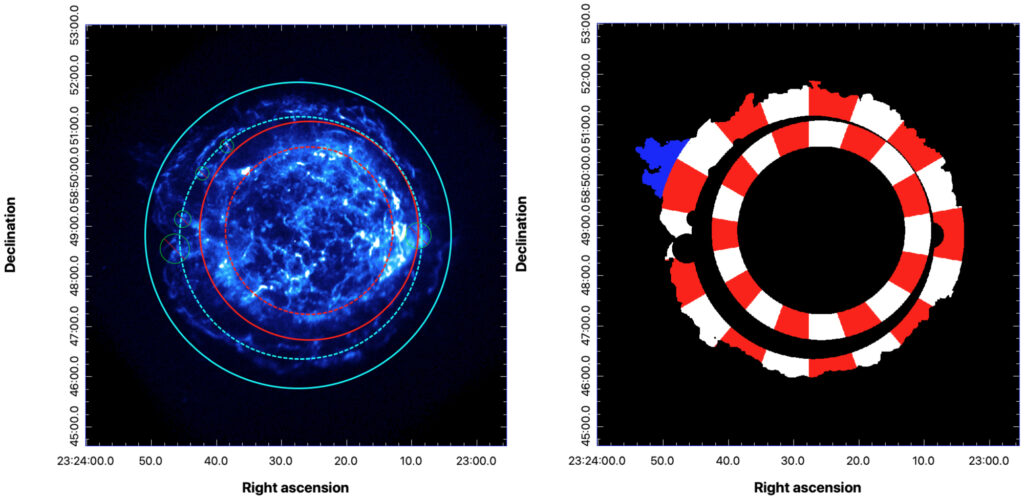
Full Article:
Vink, J. (SHARP), Patnaude, D. J. and Castro, D. (2022). The Forward and Reverse Shock Dynamics of Cassiopeia A. The Astrophysical Journal, 929, doi: 10.3847/1538-4357/ac590f
License: CC BY 4.0