Using ion tracing in a model shock front we study heating of thermal (Maxwellian) and superthermal (Vasyliunas–Siscoe) populations of protons, singly charged helium, and alpha particles. It is found that heating of thermal and superthermal populations is different, mainly because of substantially higher ion reflection in the superthermal populations. Accordingly, the temperature increase of initially superthermal populations is substantially higher than that of the thermal ions. Heating per mass decreases with the increase of the mass-to-charge ratio because of the reduced effect of the cross-shock potential and, accordingly, weaker ion reflection. The findings are supported by two-dimensional hybrid simulations.
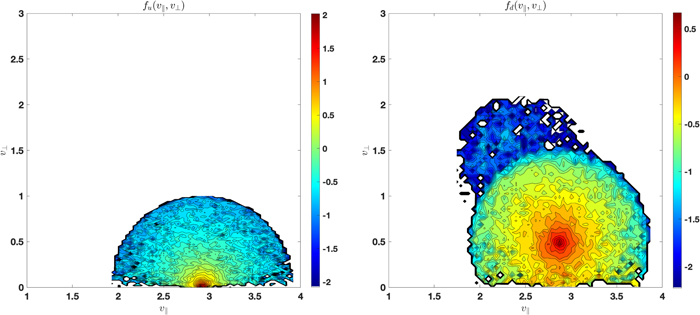
Full Article:
Gedalin, M. (SHARP), Roytershteyn, V., Pogorelov, N. V. (2023). Shock Heating of Incident Thermal and Superthermal Populations of Different Ion Species. The Astrophysical Journal, 945, doi: 10.3847/1538-4357/acb13a
License: CC BY 4.0